How to Tackle MATLAB Assignments on Electric Vehicle (EV) Power Supply System Design
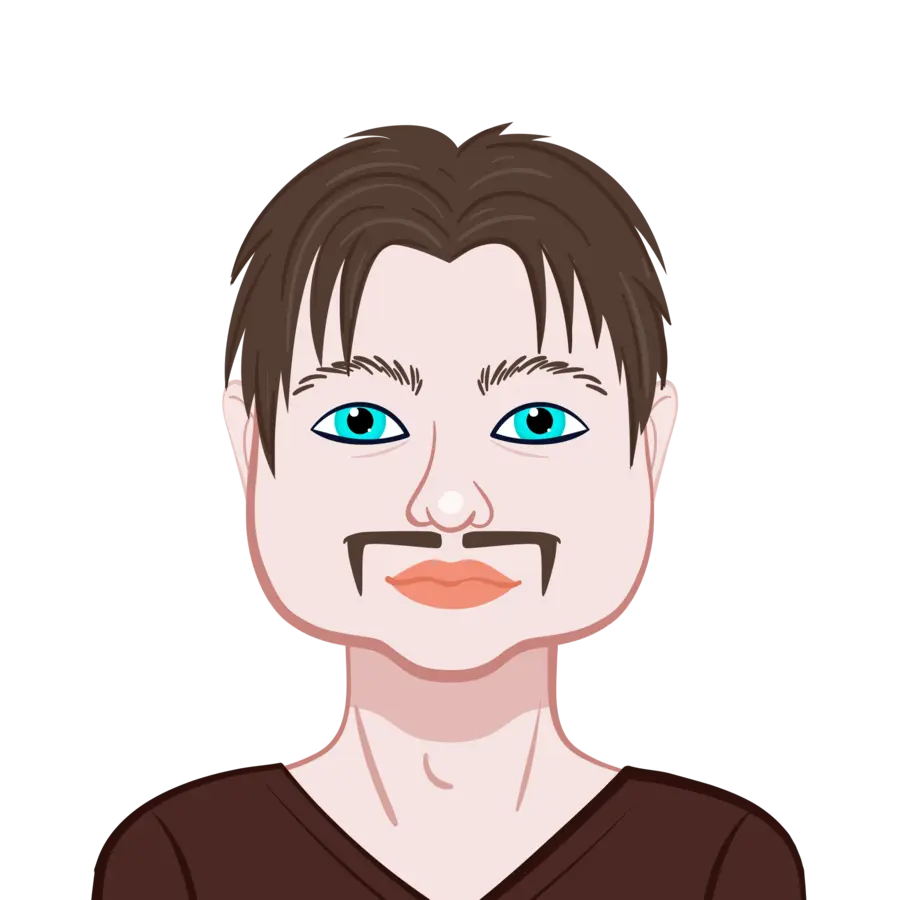
The shift toward electric vehicles (EVs) is one of the most significant trends in sustainable transportation today. As environmental concerns and fuel prices push the world towards cleaner mobility solutions, EVs are increasingly becoming the focus of both academic research and industrial development. One of the key elements in making EVs viable is the development of efficient power supply systems—particularly fast charging stations that can reliably and safely deliver high levels of power to the battery packs.
Engineering students, especially those studying electrical or power electronics, are now often required to simulate and design such systems using MATLAB and Simulink. These assignments not only test their technical understanding but also their ability to apply theoretical knowledge to real-world problems.
This blog serves as a comprehensive guide for students who need help to solve your MATLAB assignment effectively. While it’s inspired by typical EV power supply tasks, it is not limited to one specific assignment and instead focuses on a general approach that can be used across similar types of projects.
Understanding the System Requirements
Before diving into design or simulations, it’s essential to fully understand the system you're expected to model. Most EV charging system assignments will ask you to work on a complete power conversion chain—from the AC power source to the DC supply that charges the EV battery. The system may consist of components like:
- A standard AC power source (typically 230V or 400V, at 50Hz)
- A full-wave rectifier to convert AC to DC
- One or more DC-DC converters to adjust voltage levels for different subsystems
- Control units that may require separate power supply levels
- The final load, which is generally the battery pack of an electric vehicle
Understanding this basic architecture allows you to plan your simulation model in MATLAB or Simulink effectively. If provided, a block diagram can significantly help in identifying the different functional blocks and how they connect to each other.
Step 1: AC to DC Conversion – Designing the Rectifier Stage
The first active stage in most EV power supply systems is the AC to DC conversion. This is usually done with a full-wave rectifier, which ensures that both halves of the AC waveform are used to produce a continuous DC output. Students are typically asked to design a rectifier circuit and simulate it under specific load and source conditions.
To start the design, you need to account for values such as:
- Source resistance and inductance
- Load resistance and inductance
- Desired output power
In MATLAB Simulink, this stage can be built using standard blocks like “Diode,” “AC Voltage Source,” and “Series RLC Branch.” You may also need to insert a filter stage, typically a capacitor or LC filter, to smooth out the ripple in the rectified output.
When designing this part of the system, always ensure that the selected diodes can handle the expected peak inverse voltage and current. Once the rectifier is built, simulate its behavior and observe key outputs like the DC voltage waveform, ripple, and total harmonic distortion. Performance metrics such as form factor, ripple factor, and efficiency should be calculated and interpreted in the context of power quality.
Step 2: Designing a Low-Voltage DC-DC Converter for Control Systems
Control units in EV systems often require a low, stable voltage level—commonly around 30V. Designing a DC-DC converter for this purpose involves choosing between buck, boost, or buck-boost converters based on the input-output voltage relationship.
Once the topology is selected, calculate the inductor and capacitor values required to maintain output stability and keep the voltage ripple within specified limits. The ripple is usually constrained to less than 5% of the output voltage for sensitive control systems.
Another important design aspect is the choice of switching frequency. A higher frequency allows for smaller passive components but can lead to greater switching losses. You’ll need to strike a balance based on efficiency and component availability.
In Simulink, you can model the converter using IGBT or MOSFET switches, controlled through PWM signals. After simulation, analyze the output voltage and current waveforms, and confirm that the voltage ripple meets the desired constraints. Provide justifications for your component choices and explain any design trade-offs made during the process.
Step 3: Designing a High-Voltage DC-DC Converter for EV Battery Charging
Charging an electric vehicle battery requires a high voltage output—often in the range of hundreds of volts. In many assignments, you may be required to design a DC-DC converter with a 500V output. This part of the assignment typically involves designing a boost converter or other suitable topology that can step up the rectified DC voltage to the required level.
As with the control system converter, begin by calculating the required values for the inductor, capacitor, and switching frequency. The output ripple requirement here is often looser than for the control system (up to 10%), but still must be strictly maintained.
You must also consider real-world factors like component ratings, thermal limits, and protection requirements. Simulating this system in MATLAB will help verify if your theoretical calculations hold true under actual load conditions. Observe the transient and steady-state performance of the system, and ensure the output voltage is regulated appropriately.
In the report or documentation, provide a clear explanation of the converter topology chosen, the reasoning behind component values, and insights from the simulation results.
Step 4: Integrating and Simulating the Entire System
Once all individual blocks are functioning properly, it’s time to integrate them into a single simulation model. This is a critical step, as it validates the performance of your system as a whole.
The integrated model should include:
- The AC supply and rectifier
- The two DC-DC converters
- The control system and EV load
You should simulate the behavior of the system under different power levels, typically ranging from partial load (e.g., 10kW) to full load (50kW). The output current of the EV charger should be adjusted based on these load requirements.
During simulation, analyze the efficiency of the entire system. This includes calculating input and output power at various points and observing how much energy is lost in conversion. Also assess the harmonic distortion in the input current using Fourier analysis tools available in MATLAB, and determine the system's power factor.
The aim here is not just to verify functionality, but also to understand performance limits and suggest possible improvements. For instance, if harmonic distortion is too high, you might consider using active filters or modifying the switching scheme.
Step 5: Understanding the Role of Embedded Systems in Power Electronics
Finally, most advanced EV charger assignments require some knowledge of embedded systems. These are microcontroller-based systems used to implement the control logic for power converters. In practical terms, they receive sensor data (like current and voltage levels), process it, and generate PWM signals for switches.
Start by understanding the core components of an embedded system: the processor, memory units, input/output interfaces, and the software algorithm. These components must work together in real time to ensure the converter operates safely and efficiently.
Students should also become familiar with various types of embedded systems—standalone systems for simple applications, real-time embedded systems for fast response applications, and networked embedded systems used in advanced automotive electronics.
In MATLAB/Simulink, Stateflow can be used to simulate basic embedded control logic. The embedded system's response can be tested by introducing load disturbances or setpoint changes, and observing how quickly and accurately the system reacts.
This part of the assignment usually involves some theoretical understanding of embedded architectures, along with a basic block diagram showing how components like ADCs, timers, and digital outputs connect in a real-world setup.
Conclusion
Assignments involving EV power supply systems can seem complex at first, but they are a valuable opportunity to bridge the gap between theory and practical engineering. By breaking the assignment into clear stages—rectification, DC-DC conversion, system integration, and control—you can systematically tackle the problem using MATLAB and Simulink.
Focus on understanding the purpose of each subsystem, perform detailed calculations, and validate your designs through simulation. When possible, explore performance enhancements and think critically about how your system might behave in real-world conditions.
Finally, always document your work carefully, include visuals from simulations, and justify every major design decision. This not only helps in academic evaluation but also trains you in how engineers present and defend their designs in industry.
Whether you’re working on a university assignment or preparing for a career in EV systems, mastering these concepts will place you ahead in the field of power electronics and sustainable energy systems.